The Raspberry Pi is fast enough to be used to directly interface to 1-Wire bus without the need for drivers. The advantages of programming our own 1-wire bus protocol is that it doesn't depend on the uncertainties of a Linux driver.
Now On Sale!
You can now buy a print or ebook edition of Raspberry Pi IoT in C from Amazon.
For Errata and Listings Visit: IO Press
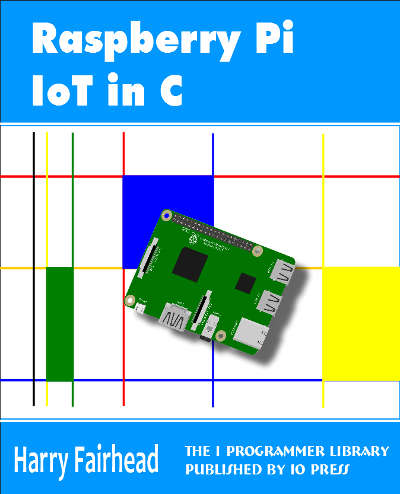
This our ebook on using the Raspberry Pi to implement IoT devices using the C programming language. The full contents can be seen below. Notice this is a first draft and a work in progress.
Chapter List
-
Introducing Pi (paper book only)
-
Getting Started With NetBeans In this chapter we look at why C is a good language to work in when you are creating programs for the IoT and how to get started using NetBeans. Of course this is where Hello C World makes an appearance.
-
First Steps With The GPIO
The bcm2835C library is the easiest way to get in touch with the Pi's GPIO lines. In this chapter we take a look at the basic operations involved in using the GPIO lines with an emphasis on output. How fast can you change a GPIO line, how do you generate pulses of a given duration and how can you change multiple lines in sync with each other? -
GPIO The SYSFS Way
There is a Linux-based approach to working with GPIO lines and serial buses that is worth knowing about because it provides an alternative to using the bcm2835 library. Sometimes you need this because you are working in a language for which direct access to memory isn't available. It is also the only way to make interrupts available in a C program. -
Input and Interrupts
There is no doubt that input is more difficult than output. When you need to drive a line high or low you are in command of when it happens but input is in the hands of the outside world. If your program isn't ready to read the input or if it reads it at the wrong time then things just don't work. What is worse is that you have no idea what your program was doing relative to the event you are trying to capture - welcome to the world of input. -
Memory Mapped I/O
The bcm2835 library uses direct memory access to the GPIO and other peripherals. In this chapter we look at how this works. You don't need to know this but if you need to modify the library or access features that the library doesn't expose this is the way to go. -
Near Realtime Linux
You can write real time programs using standard Linux as long as you know how to control scheduling. In fact it turns out to be relatively easy and it enables the Raspberry Pi to do things you might not think it capable of. There are also some surprising differences between the one and quad core Pis that make you think again about real time Linux programming. -
PWM
One way around the problem of getting a fast response from a microcontroller is to move the problem away from the processor. In the case of the Pi's processor there are some builtin devices that can use GPIO lines to implement protocols without the CPU being involved. In this chapter we take a close look at pulse width modulation PWM including, sound, driving LEDs and servos. -
I2C Temperature Measurement
The I2C bus is one of the most useful ways of connecting moderately sophisticated sensors and peripherals to the any processor. The only problem is that it can seem like a nightmare confusion of hardware, low level interaction and high level software. There are few general introductions to the subject because at first sight every I2C device is different, but here we present one. -
A Custom Protocol - The DHT11/22
In this chapter we make use of all of the ideas introduced in earlier chapters to create a raw interface with the low cost DHT11/22 temperature and humidity sensor. It is an exercise in implementing a custom protocol directly in C. -
One Wire Bus Basics
The Raspberry Pi is fast enough to be used to directly interface to 1-Wire bus without the need for drivers. The advantages of programming our own 1-wire bus protocol is that it doesn't depend on the uncertainties of a Linux driver. -
iButtons
If you haven't discovered iButtons then you are going to find of lots of uses for them. At its simples an iButton is an electronic key providing a unique coce stored in its ROM which can be used to unlock or simply record the presence of a particular button. What is good news is that they are easy to interface to a Pi. -
The DS18B20
Using the software developed in previous chapters we show how to connect and use the very popular DS18B20 temperature sensor without the need for external drivers. -
The Multidrop 1-wire bus
Some times it it just easier from the point of view of hardware to connect a set of 1-wire devices to the same GPIO line but this makes the software more complex. Find out how to discover what devices are present on a multi-drop bus and how to select the one you want to work with. -
SPI Bus
The SPI bus can be something of a problem because it doesn't have a well defined standard that every device conforms to. Even so if you only want to work with one specific device it is usually easy to find a configuration that works - as long as you understand what the possibilities are. -
SPI MCP3008/4 AtoD (paper book only)
-
Serial (paper book only)
-
Getting On The Web - After All It Is The IoT (paper book only)
-
WiFi (paper book only)
The Maxim 1-Wire bus is a proprietary bus that is very easy to use and has a lot of useful devices you can connect to it including the iButton security devices. However, probably the most popular of all 1-wire devices is the DS18B20 temperature sensor - it is small, very cheap and very easy to use. The next two chapters show you how to use both of these but first let's deal with the general techniques needed to work with the 1-wire bus.
There are 1-wire drivers for Linux and these are most commonly used in Python and other programs that work with the bus. However to make these work you have to install the drivers and occasionally there are compatibility problems. If you are programming in C then you have more than enough speed to write your own 1-wire protocol functions and this has the advantage of not requiring any interaction with Linux to work. You are also free to modify and extend the 1-wire functions without having to become involved in writing Linux modules.
The Hardware
One wire devices are very simple and only use a single wire to transmit data - hence the name.
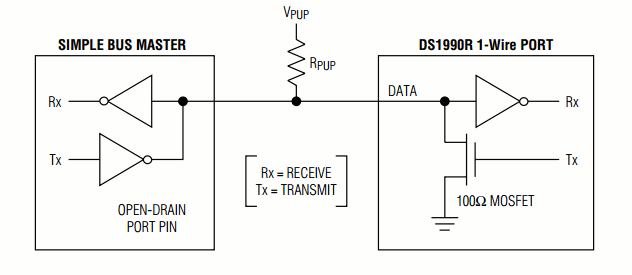
The 1-wire device can pull the bus low using its Tx line and can read the line using its Rx line. The reason for the pull up resistor is that both the bus master and the slave can pull the bus low and it will stay low until they both release the bus.
The device can even be powered from the bus line by drawing sufficient current through the pull up resistor - so called parasitic mode. Low power devices work well in parasitic mode but some devices have such a heavy current draw that the master has to provide a way to connect them to the power line - so called strong pull up. In practice parasitic mode can be difficult to make work reliably for high power devices.
In normal powered mode there are just three connections
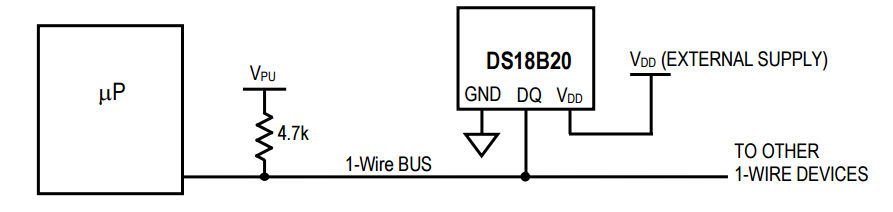
V power - usually 3.3V for the Pi
Ground
and
Data
The pull up resistor varies according to the device but anything from 2.2K to 4.7K Ohms works.
There can be multiple devices on the bus and each one has a unique 64-bit lasered ROM code, which can be used as an address to select the active devices.
For simplicity, it is better to start off with a single device and avoid the problem of enumerating the devices on the bus - although once you know how everything works this isn't difficult to implement.
So to get started select a 1-wire device that you want to work with and set it up ready to talk to the Pi of your choice. In the next chapter we show how to work with an iButton and in the following chapter the DS18B20 is explained. The functions described in this chapter should work with any 1-wire device.
Initialization
Every transaction with the a 1-wire device starts with an initialization handshake.
First we have to work out how to configure the GPIO line. This example assumes that the 1-wire device is connected to pin 7. If this isn't the case change the pin enumeration to the correct pin. For a full practical example see either of the next two chapters.
You might think that we have to initialize the GPIO line so that it works in pull up mode.
This isn't necessary and the default push pull mode will do.
The reason is that in the case of the 1-wire bus the master controls when other devices send their data. Typically the master sends a pulse and then the slaves respond by pulling the line low. As long as the master doesn't drive the line during the period when the slaves are responding everything is fine.
What we do in practice is to configure the GPIO line for output only when the master needs to drive the line. Once the master is finished the GPIO line is set back to input and the pull up resistor is allowed to pull the line back up. After this any slave wanting to send data is free to pull the line low.
The first transaction we need is the initialization pulse.
This is simply a low pulse that lasts at least 480 microseconds, a 15 to 60 microsecond pause follows and then any and all of the devices on the bus pull the line low for 60 to 240 microseconds.
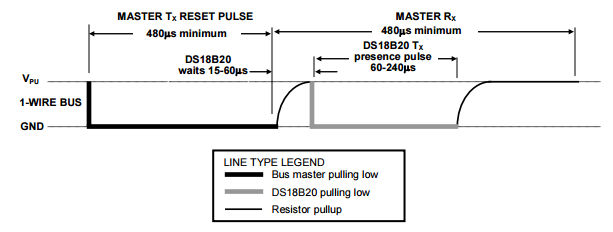
The suggested timings are:
low for 480 microseconds and read the line after 70 microsecond followed by a 410 pause.
This is fairly easy to implement as a function:
int presence(uint8_t pin) { bcm2835_gpio_fsel(pin, BCM2835_GPIO_FSEL_OUTP); bcm2835_gpio_write(pin, LOW); bcm2835_delayMicroseconds(480); bcm2835_gpio_fsel(pin, BCM2835_GPIO_FSEL_INPT); bcm2835_delayMicroseconds(70); uint8_t b = bcm2835_gpio_lev(pin); bcm2835_delayMicroseconds(410); return b; }
We pull the line low for 480 microseconds and then let it be pulled back up by changing the line to input i.e. high impedance. After a 70 microsecond wait which is right at the start of the guaranteed period when the line should be low if there is an active device on the bus we read the input line and then wait another 410 microseconds to complete the data slot.
The timings in this case are not critical as long as the line is read while it is held low by the slaves - which is never less than 60 microseconds and is typically as much as 100 microseconds.
The actual pulse timings with the values given are 483 microsecond reset and a total slot time of 632 microseconds.
If there is a device the function should return zero and if there are no devices it should return a one.
if(presence(RPI_GPIO_P1_07)==1){
printf("No device \n");
}
If you try this partial program and have a logic analyzer with a 1-wire protocol analyzer you will see something like:

Seeing a presence pulse is the simplest and quickest way to be sure that your hardware is working.
- Prev
- Next >>